Bone Substitutes in Oral Implantology
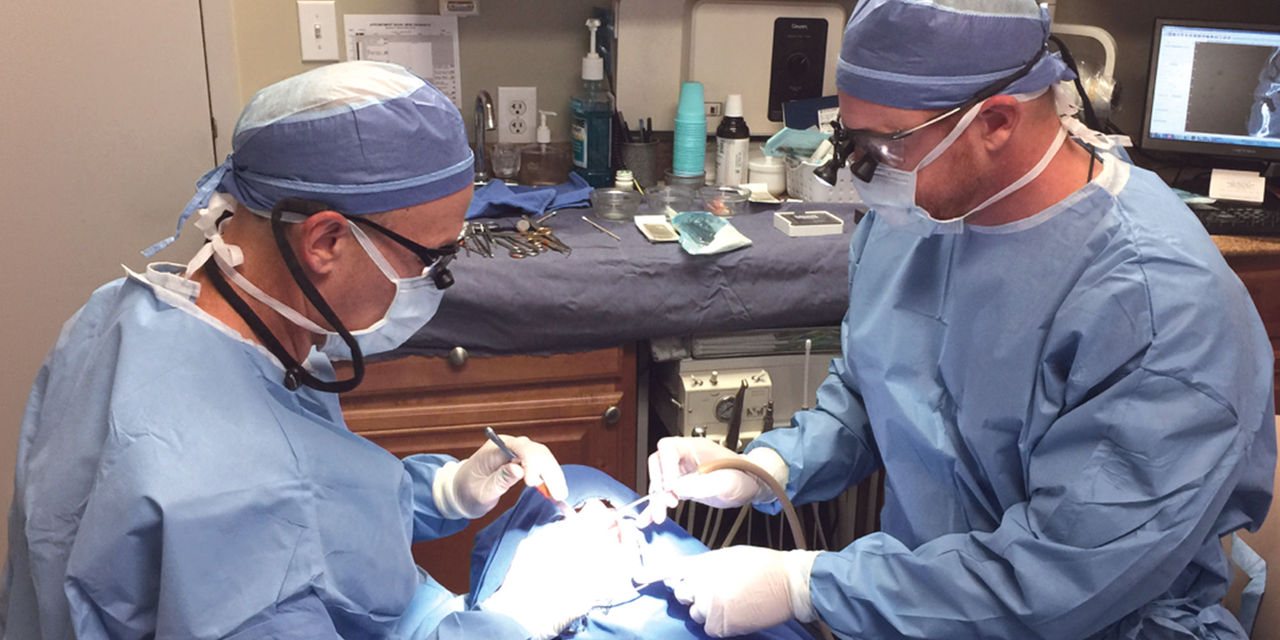
Adequate volume of available bone is one of the most important prerequisites for predictable implant placement and osseointegration. Although loss in bone volume may result from trauma, bone deficiency is most frequently due to the normal physiologic process that occurs after tooth loss or extraction. Studies have shown that resultant bone resorption after tooth removal can be approximately 1.5–2 mm vertically and 3.8 mm in the horizontal plane within six months.1,2 Restoring this loss of bone volume is paramount for establishing an ideal site for implant placement.
Today, bone regeneration procedures are widely accepted as a viable option for the treatment of edentulous spaces to be restored with an implant-supported prosthesis. Implant clinicians have a wide range of bone grafting materials and procedures at their disposal. For years, the gold standard in bone regeneration has been the use of autogenous (autograft) bone because of its inherent osteoconductive, osteoinductive and osteogenic properties. Because autogenous bone is composed of the patient’s own tissue, there is a reduction in the likelihood of immunoreactions and possible infectious transmission. However, autogenous bone grafting has many disadvantages, including the need for a secondary surgical site, potential increase in pain and discomfort, quantity restrictions, increased costs and longer surgical procedures. Studies have shown that only 61 percent of patients accept grafting of autogenous origin.3
IDEAL CHARACTERISTICS OF BONE GRAFT MATERIAL
- Biocompatibility
- Bioactive to promote cell differentiation and proliferation
- Low incidence of infection
- Nontoxic and non-immunogenic
- Ability to maintain space and volume over time
- Ability to be replaced entirely with new bone growth
- Resorption rate to coincide with bone formation
Because of these shortcomings, the use of bone substitutes has become extremely popular in implant dentistry. The ideal characteristics of a bone substitute include biocompatibility, low incidence of infection and immunogenicity, maintenance of space and volume over time, and the ability to be replaced entirely with new, viable bone growth. In order to comprehend the concept of bone regeneration and to select the ideal bone graft material, the implant clinician should have a strong understanding of bone biology.
BONE BIOLOGY
Mechanisms of Bone Healing
Bone is a dynamic biological tissue composed of metabolically active cells that are influenced by biochemical, biomechanical, cellular, hormonal and pathologic mechanisms. As such, there are various mechanisms of the bone deposition, resorption and remodeling process.
Bone is a dynamic biological tissue composed of metabolically active cells that are influenced by biochemical, biomechanical, cellular, hormonal and pathologic mechanisms.
- Bone remodeling involves the replacement of old bone tissue with new bone tissue, which occurs naturally to maintain healthy bone mass.
- Bone modeling — also known as Wolff’s law — is the process by which bone structure adapts to loading by changing shape, curvature and mass.
- Bone repair is a proliferative physiological process that results in the healing of bone after trauma has occurred.
- Bone regeneration is the process that utilizes surgical protocols that enable bone growth in deficient sites using principles of osteogenesis, osteoinduction or osteoconduction.
Macroscopic and Microscopic Features of Bone
When evaluating bone grafting materials, the macroscopic and microscopic features of bone must be understood. Macroscopically, bone is composed of cortical or cancellous bone. Cortical, or compact, bone describes the dense bone encompassing the maxilla and mandible. Cortical bone is composed of mineralized matrix, is considered load-bearing, and contains relatively few cells and blood vessels. The primary and functional unit of cortical bone is the osteon, or haversian system, which consists of concentric layers of compact bone tissue surrounding a central canal.
In contrast, cancellous, or trabecular, bone is less dense and is found between the cortical plates of the maxilla and mandible. Cancellous bone varies in density and is composed of thick and thin trabeculae. Although cancellous bone has a higher surface area-to-mass ratio than cortical bone, it is much weaker and less dense. The primary functional unit of cancellous bone is the trabecula. The trabeculae maintain a mechanical function that lessens tensile forces; however, their mass is usually reduced as age increases.
Microscopically, bone is classified as either lamellar or woven bone. Woven bone is highly cellular, less organized, poorly mineralized, and the type of bone that forms immediately adjacent to an implant site after placement. Lamellar bone is highly organized load-bearing bone and is arranged in concentric haversian systems. Ideally, lamellar bone is responsible for osseointegration around a dental implant.
Bone Cell Types
Four types of bone cells are involved in the bone regeneration process:
- Osteoprogenitor cells — also termed undifferentiated stem cells, pluripotential cells, stem cells and bone marrow stromal cells — are located within the inner cellular layer of the periosteum, and undergo mitotic division and differentiate into osteoblasts during the bone regeneration process.
- Osteoblasts are the bone-forming cells that are responsible for deposition and calcification of the extracellular bone matrix. Osteoblasts are initially derived from mesenchymal stem cells in the bone marrow. Mature osteoblasts are responsible for synthesizing collagen and other proteins, such as growth factors, and eventually become known as “resting surface cells” or osteocytes.
- Osteocytes are mature, fully differentiated osteoblasts that have been surrounded by mineralized bone matrix, and act as mechanoreceptors of bone and regulators of the turnover of bone.
- Osteoclasts are the cells responsible for the resorption of bone in the modeling and remodeling process. They are large, multinucleated cells that utilize enzymes, including collagenase, lysosomal enzymes and acids, for the resorption of mineralized bone matrix and collagen degradation. The actions of osteoclast cells are controlled by hormones, including PTH, D3, calcitonin, glucocorticoids, prostaglandins, ILGF, TNF, TGF-β, androgens, thyroid hormones and bisphosphonates, as well as by local factors such as the vascular system, stress and strain.
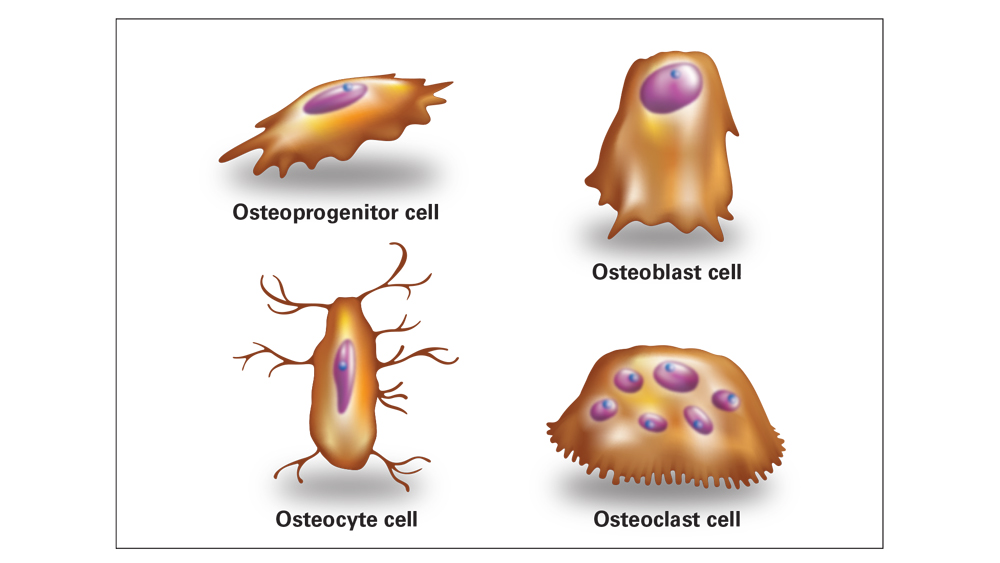
Bone regeneration involves four types of bone cells. Osteoprogenitors are stem cells that differentiate into osteoblasts; osteoblasts are responsible for producing bone matrix; osteocytes are mature bone cells responsible for bone maintenance; and osteoclasts are responsible for the resorption of bone in the modeling and remodeling processes.
Osteoregeneration
Osteoregeneration involves three important biological properties: osteoconduction, osteoinduction and osteogenesis.
- Osteogenesis describes the natural process of bone formation within the body. Osteogenesis results in new bone formation by live, viable cells derived from the host.
- Osteoinduction allows the graft to induce the primitive, undifferentiated and pluripotent cells to develop into the bone-forming cells, thus inducing osteogenesis.
- Osteoconduction refers to the ability of osteoblasts and osteoprogenitor cells to migrate and grow within the scaffold or framework (three-dimensional architecture) of the graft. Healthy host bone must be present and in contact with the bone graft for successful osteoconduction to occur.
BONE GENERATION
- Osteogenesis: bone formation from live, viable cells
- Osteoinduction: graft material induces bone formation
- Osteoconduction: graft material provides a scaffold of bone growth
GRAFT MATERIAL OPTIONS
I. Allograft Bone
Allogenic bone is harvested from an individual of the same species and transplanted to a genetically different person. Allografts are considered to be the most ideal alternative to autografts. Allografts are available in many different preparations, with the most common being freeze-dried bone allografts (FDBA) and demineralized freeze-dried bone allografts (DFDBA). Although their biological properties vary, overall they exhibit osteoconductive qualities with reduced osteoinductive properties. FDBA and DFDBA possess the advantage of decreased morbidity to the patient, as there is no second surgical site.
Allografts are considered to be the most ideal alternative to autografts.
Allografts undergo extensive and rigorous processing procedures. First, allografts are processed by freeze-drying at approximately -15 to -20 degrees Celsius, which allows for easier handling with a decreased antigenicity. The main drawbacks of freeze-dried bone include the potential risk of cross-infection and the possibility of immunologic reactions due to its protein content. The possibility of disease transmission cannot be completely eliminated; however, there are no documented cases of disease transmission from the use of allografts in dentistry, including over one million cases over a 25-year period.4 Additionally, allografts exhibit an inconsistent quality because the age and the health of the donor affect the bone regeneration properties of the material. Allografts are primarily osteoconductive materials, with a reduced osteoinductivity present with demineralized bone matrix (DBM) preparations.
INDICATIONS FOR MINERALIZED FDBA
- Socket preservation
- Guided bone regeneration (GBR) ridge augmentation
- Maxillary sinus augmentation
- Treatment of peri-implant defects
Types of Allografts
The most common allografts used in implant dentistry have varying characteristics.
- Demineralized freeze-dried bone allograft (DFDBA) is osteoconductive and osteoinductive. The material consists of highly processed bone with at least 40 percent of the mineral content of the bone matrix removed by 0.5–0.6 M hydrochloric acid until the calcium content is reduced to less than 2 percent.5 This allows for increased availability of matrix-associated bone morphogenetic proteins (BMP) or growth factors that allow the graft to become osteoinductive.
- Freeze-dried bone allograft (FDBA) is an allogenic bone that does not undergo the demineralization process. Also referred to as “mineralized” because the mineral content has not been reduced, FDBA has the same BMP content in its organic matrix. However, it does not have the same osteoinductive capability as DFDBA. FDBA has been shown to be a better scaffold for osteoconduction than DFDBA, which allows for superior space maintenance.6 Eventually, osteoclasts break down the mineral content of FDBA until demineralization occurs, which induces new bone formation and a prolonged protein release.
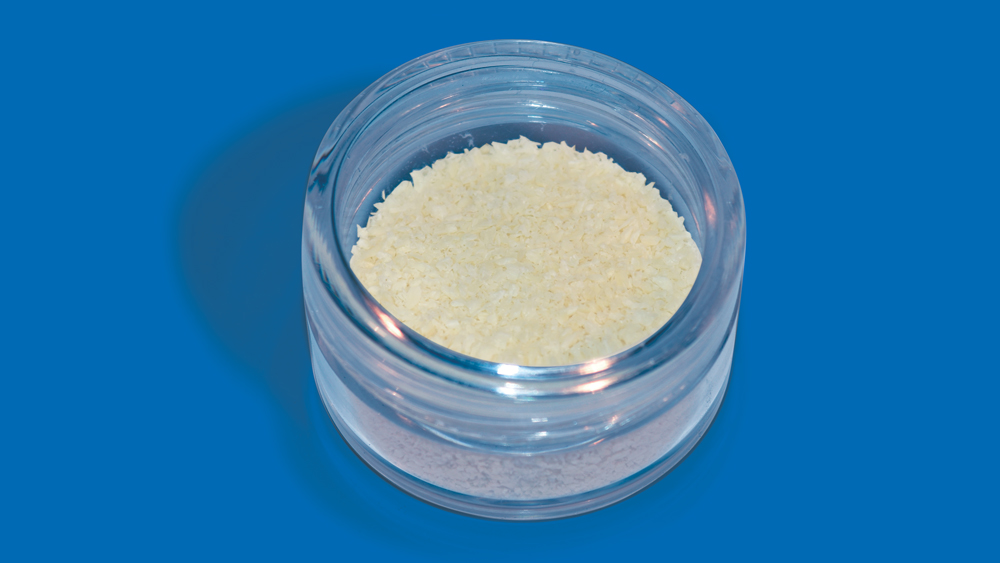
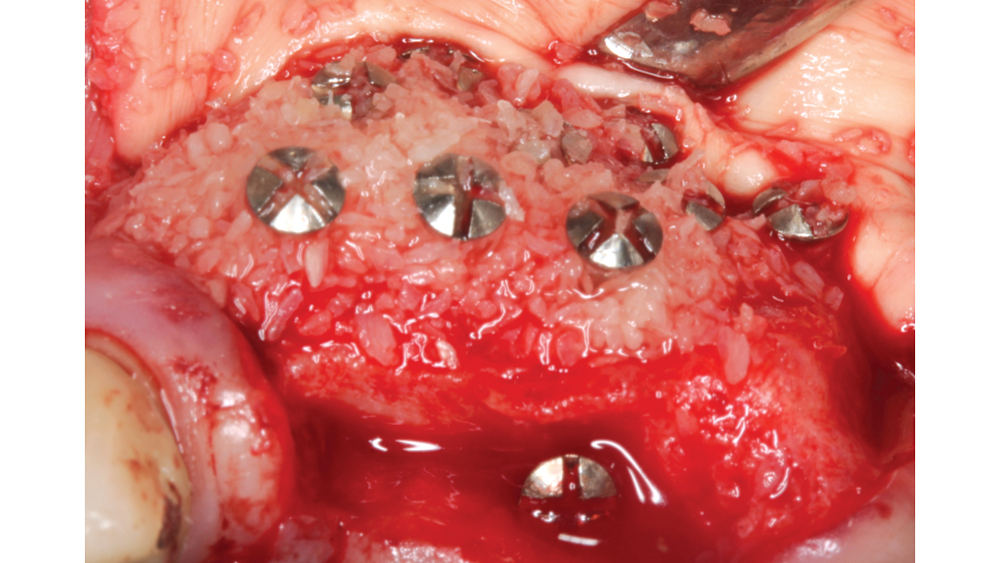
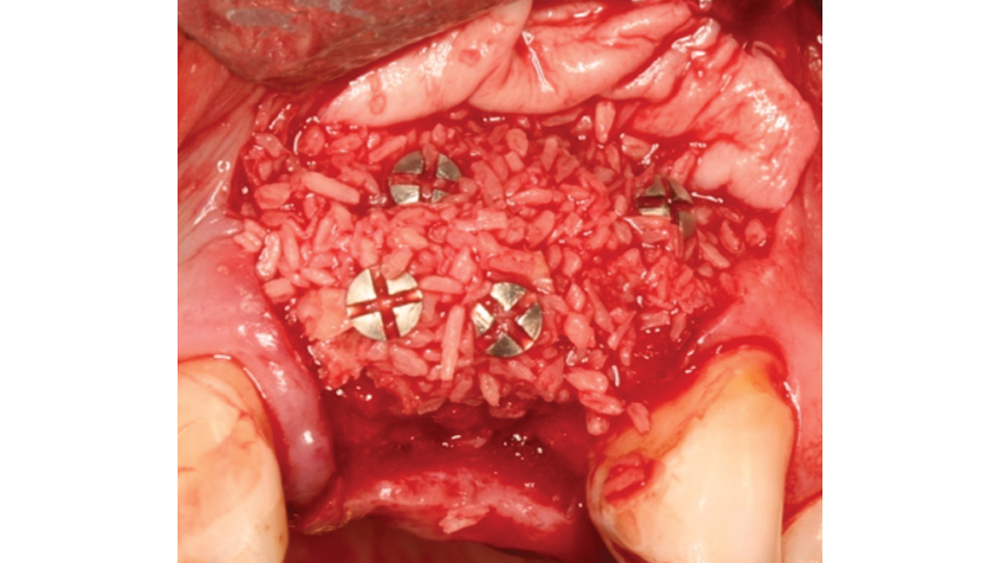
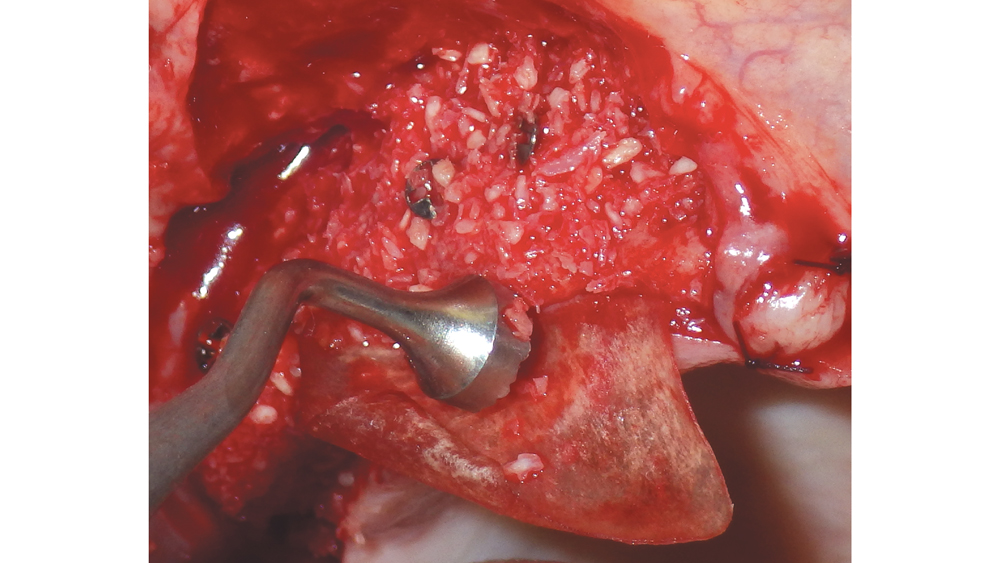
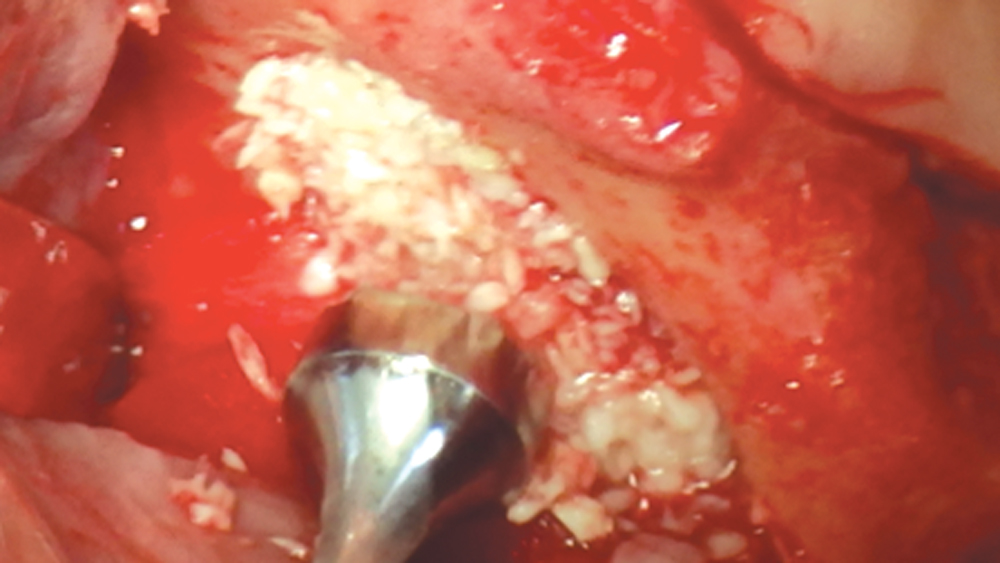
Newport Biologics™ Mineralized Cortico/Cancellous Allograft Blend (Glidewell Direct; Irvine, Calif.) possesses the optimal features of cortical and cancellous bone, with particle sizes that allow for rapid site revascularization and increased structural integrity. The cancellous bone allows for better cell migration and faster remodeling, and the cortical bone provides the space maintenance that allows the time for bone regeneration to occur. The particle size is approximately 250–1,000 microns, with a turnover rate of 4–6 months for predictable bone regeneration. Shown are various clinical GBR and lateral ridge augmentation procedures with the use of mineralized cortico/cancellous bone.
Particle Form and Size
The allograft particle form and size contribute to the predictability of bone regeneration.
- Ideal particle form — Allografts are available in three particle forms: cortical, cancellous and cortico/cancellous. Cortical allografts are associated with an increased density and greater space maintenance properties, which allow for slower resorption. Cancellous chips are advantageous because they allow for osteoconductive scaffolding and deposition of osteoblasts while also providing faster resorption. The cortico/cancellous mixture allows for the benefits of both cancellous and cortical bone.
The allograft particle form and size contribute to the predictability of bone regeneration. … The cortico/cancellous mixture allows for the benefits of both cancellous and cortical bone.
- Ideal particle size — The particle size of the allograft material is very important in the bone regeneration process, as a particle size that is too small (less than 125 microns) leads to fast resorption with inconsistent bone formation. A larger particle size (greater than 1,000 microns) restricts resorption and may be sequestered or result in delayed healing. Studies have shown an ideal particle size for predictable bone regeneration to be approximately 250–1,000 microns.7
II. Xenografts
Xenografts are bone grafts originating from a different species. Most commonly, xenografts are derived from bovine (cattle) origins, with less common sources including equine (horses) and porcine (pigs). The most common xenografts are natural hydroxyapatite (HA) derived from animal bone and anorganic bone matrix produced from bovine sources. Xenogenic bone grafts exhibit excellent osteoconductive properties and act as a scaffold for newly deposited bone. Although xenografts are available in greater supply than allograft materials, they have been shown to exhibit elevated inflammatory responses along with a very slow and inconsistent resorption process. Consideration must also be given to the risk of cross-contamination with bovine spongiform encephalopathy or porcine endogenous retroviruses. Unfortunately, it has been shown to be very difficult to adequately screen xenografts for the possibility of viral presence.8
III. Alloplasts
Because of the possibility of disease transmission from allografts and xenografts, there has recently been an increased demand for other bone substitute options. Alloplasts, which are synthetic, are a biocompatible option for the implant clinician. Alloplasts have the advantages of no immunogenic response and no risk of disease transmission. These materials have been shown to be osteoconductive, with an interconnecting pore system that serves as a scaffold for the migration of bone-forming cells.9
Types of Alloplasts
A wide range of synthetic materials have been developed, such as synthetic HA, β-tricalcium phosphate (β-TCP), calcium-phosphate cements, and glass ceramics.
- Hydroxyapatite (HA) is the basic component of inorganic bone and exhibits a similar chemical composition to natural bone. HA is most commonly processed from natural reef coral skeletons or homogenized calcium-phosphate powder. It is biocompatible and osteoconductive, and has excellent space-maintaining qualities. However, synthetic HA has shown unpredictable and slow degradation after approximately 1–2 years.10
- Tricalcium phosphate (TCP) has a calcium-to-phosphate ratio of 1.5, which is much lower than HA and results in less compressive strength. Calcium phosphates resorb 10–20 times faster than HA, and their macroscopic mechanical properties are inadequate for load-bearing surfaces because of their inherent brittleness. Because of the fast biodegradation rate, this bone is highly unpredictable and is not consistent with adequate bone deposition.
When carbonate apatite is combined with collagen, the biological stability and strength are increased, which allows the scaffolds to act as a delivery vehicle for growth factors and living cells for bone formation.
- Carbonate apatite with collagen has been shown to resemble bone more than any other calcium phosphate available. The inorganic content of bone contains approximately 7 percent carbonate by weight.11 Studies have shown that carbonate apatite exhibits a more controlled resorptive pattern as well as excellent osteoconductivity and biocompatibility.12 When carbonate apatite is combined with collagen, the biological stability and strength are increased, which allows the scaffolds to act as a delivery vehicle for growth factors and living cells for bone formation.13 Scanning electron microscope (SEM) studies have shown that the highly porous and interconnected structure ensures a biological environment that is conducive to cell attachment, proliferation, angiogenesis and tissue growth.14
- Bioactive glasses are ceramic substitutes that are reinforced by oxides — sodium oxide, calcium oxide, phosphorus pentoxide and silicon dioxide — and exhibit questionable mechanical strength. They are absorbable and have no risk of disease transmission or immune responses. The bioactive ceramics exhibit improved mechanical properties relative to bioactive glass, but they are still brittle enough to fracture when subjected to cyclic loading. In order to improve their resistance to fracture, methods of incorporating stainless steel and zirconia fibers have been performed. Studies have shown questionable efficacy of bioactive glasses with respect to osteoconduction qualities and the ability to bond to tissues (bioactivity).15
INDICATIONS FOR BONE GRAFT PUTTY (CARBONATE APATITE + TYPE I COLLAGEN)
- Maxillary sinus augmentation
- Larger defects that require slower resorption
- High stress (muscle pull) augmentation sites where it's imperative there is no migration of the graft material
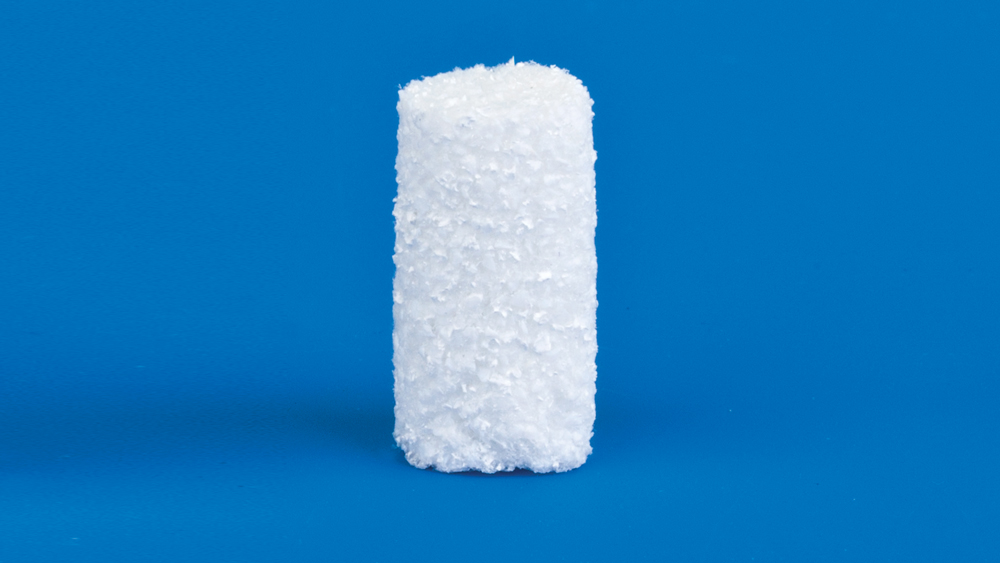
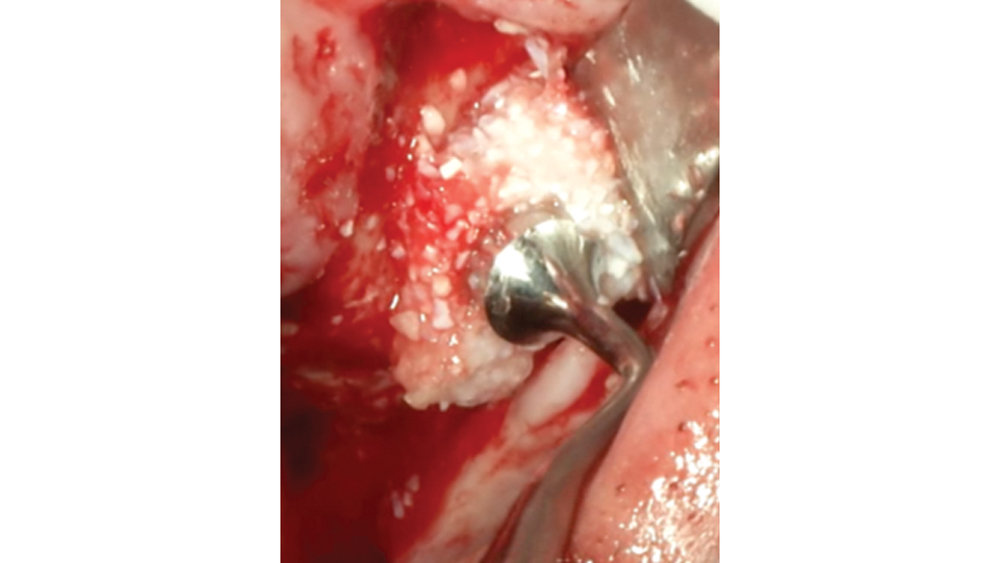
Newport Biologics Bone Graft Putty Mineral-Collagen Composite is a calcium phosphate-based grafting material that becomes moldable upon hydration. It features a carbonate apatite structure similar to natural bone, combined with a type I collagen derived from bovine Achilles tendon. The mineral particles are dispersed within collagen fibers, forming a 3D matrix that is fully resorbed during the natural process of bone formation and remodeling. This bone graft putty is ideal for sites that require no movement of the graft material, such as areas of high muscle pulls or sinus augmentation procedures. Shown is bone putty inserted into an osteotomy via the osteotome sinus graft technique.
SUMMARY
The use of bone substitutes in implant dentistry continues to be a major field of research. Allografts provide the clinician with an alternative to autogenous bone with varying degrees of osteoinductive qualities. Synthetic bone substitutes are becoming more popular because they possess no possibility of disease transmission. In deciding which bone graft material to use, the clinician must have a clear understanding of how bone heals and be able to distinguish the ideal bone material for the specific case and situation. Ideally, a bone regeneration material should be cost-effective, non-immunogenic, easily handled, and resorbed in unison with new bone formation. As more bone graft materials are discovered, newer applications and protocols will become available to help clinicians provide implant treatment in areas where deficient bone volume is present.
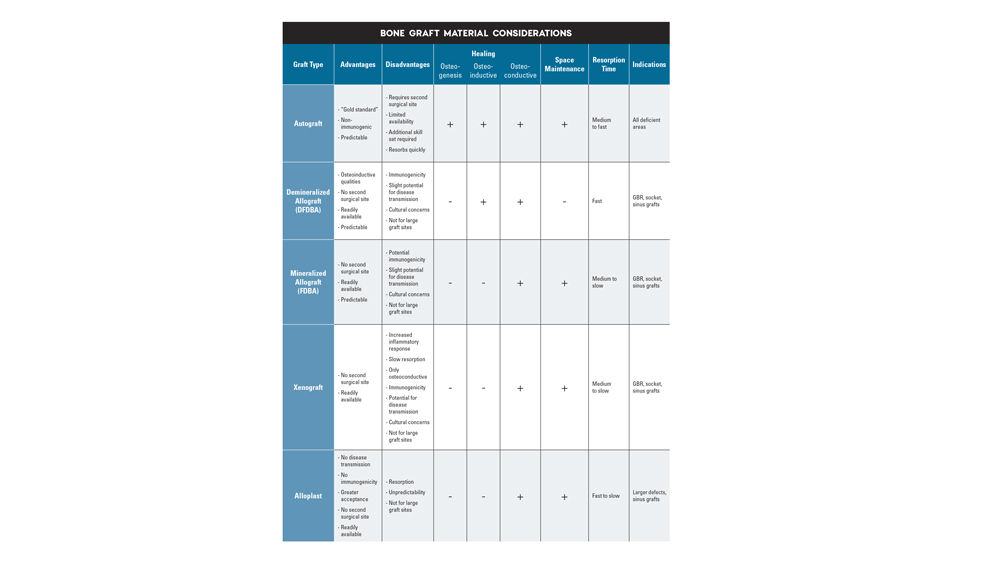
References
- ^ Liu J, Kerns DG. Mechanisms of guided bone regeneration: a review. Open Dent J. 2014 May 16;8:56-65.
- ^ Van der Weijden F, Dell’Acqua F, Slot DE. Alveolar bone dimensional changes of post-extraction sockets in humans: a systematic review. J Clin Periodontol. 2009 Dec;36(12):1048-58.
- ^ Hof M, Tepper G, Semo B, Arnhart C, Watzek G, Pommer B. Patients’ perspectives on dental implant and bone graft surgery: questionnaire-based interview survey. Clin Oral Implants Res. 2014 Jan;25(1):42-5.
- ^ Sanz M, Vignoletti F. Key aspects on the use of bone substitutes for bone regeneration of edentulous ridges. Dent Mater. 2015 Jun;31(6):640-7.
- ^ Boyce T, Edwards J, Scarborough N. Allograft bone: the influence of processing on safety and performance. Orthop Clin North Am. 1999 Oct;30(4):571-81.
- ^ Piattelli A, Scarano A, Corigliano M, Piattelli M. Comparison of bone regeneration with the use of mineralized and demineralized freeze-dried bone allografts: a histological and histochemical study in man. Biomaterials. 1996 Jun;17(11):1127-31.
- ^ Shapoff CA, Bowers GM, Levy B, Mellonig JT, Yukna RA. The effect of particle size on the osteogenic activity of composite grafts of allogeneic freeze-dried bone and autogenous marrow. J Periodontol. 1980 Nov;51(11):625-30.
- ^ Jacobsen G, Easter D. Allograft vs. xenograft: practical considerations for biologic scaffolds. Musculoskeletal Transplant Foundation. 2008.
- ^ Barrack RL. Bone graft extenders, substitutes, and osteogenic proteins. J Arthroplasty. 2005 Jun;20(4 Suppl 2):94-7.
- ^ Esposito M, Grusonvin MG, Rees J, Karasoulos D, Felice P, Alissa R, et al. Effectiveness of sinus lift procedures for dental implant rehabilitation: a Cochrane systematic review. Eur J Oral Implantol. 2010 Spring;3(1):7-26.
- ^ LeGeros RZ. Calcium phosphates in oral biology and medicine. Monogr Oral Sci. 1991;15:1-201.
- ^ Suh H, Park JC, Han DW, Lee DH, Han CD. A bone replaceable artificial bone substitute: cytotoxicity, cell adhesion, proliferation, and alkaline phosphatase activity. Artif Organs. 2001 Jan;25(1):14-21.
- ^ Peter M, Binulal NS, Nair SV, Selvamurugan N, Tamura H, Jayakumar R. Novel biodegradable chitosan-gelatin/nano-bioactive glass ceramic composite scaffolds for alveolar bone tissue engineering. Chem Eng J. 2010 Apr;158(2):353-61.
- ^ Salim S, Ariani MD. In vitro and in vivo evaluation of carbonate apatite-collagen scaffolds with some cytokines for bone tissue engineering. J Indian Prosthodont Soc. 2015 Oct-Dec;15(4):349-55.
- ^ Chan C, Thompson I, Robinson P, Wilson J, Hench L. Evaluation of Bioglass/dextran composite as a bone graft substitute. Int J Oral Maxillofac Surg. 2002 Feb;31(1):73-7.