The Use of Barrier Membranes in Implant Dentistry
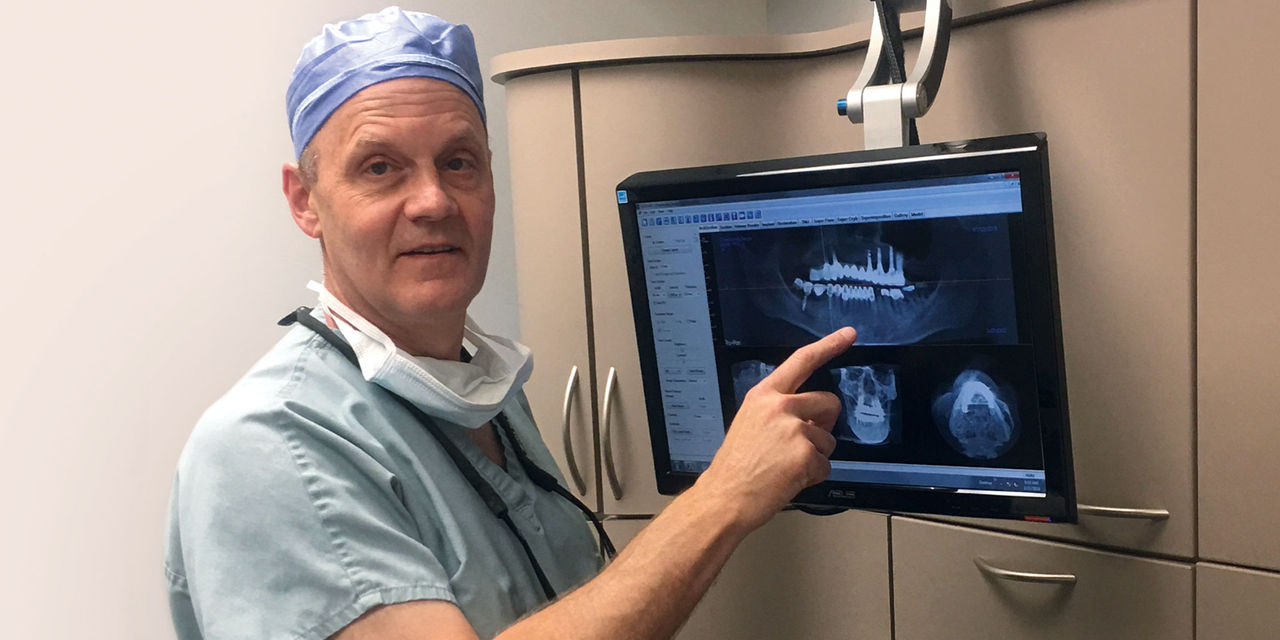
Note: The Hahn Tapered Implant System is now known as the Glidewell HT Implant System
Guided bone regeneration (GBR) is the most common technique in implant dentistry for the treatment of bony defects, and a crucial component of the GBR procedure is the use of barrier membranes. Today, there are many different membrane materials for the implant clinician to utilize depending on the clinical situation. However, it can often be overwhelming and confusing to decide which membrane is ideal to use. Therefore, this article will provide a review of the different membranes available and discuss the indications for use in various clinical situations.
WHY USE A MEMBRANE?
In general, membranes are used in GBR procedures to act as biological and mechanical barriers against the invasion of cells not involved in bone formation, such as epithelial cells, and allow for the migration of the slower-migrating bone-forming cells into the defect sites.1 As bone defects heal, there is a competition between soft-tissue and bone-forming cells to invade the surgical site. In general, soft-tissue cells migrate at a much faster rate than bone-forming cells. Therefore, the primary goal of barrier membranes is to allow for selective cell repopulation and guide the proliferation of various tissues during the healing process.2 Below the membrane, the regeneration process occurs, which involves angiogenesis and migration of osteogenic cells. The initial blood clot is replaced by woven bone after vascular ingrowth, which later is transformed into load-bearing lamellar bone. This ultimately supports hard- and soft-tissue regeneration.3 If a barrier membrane is not utilized, lack of space maintenance will result in soft-tissue integration and compromised bone growth.
Ideal Characteristics of Membrane Material
✓ Tissue compatibility
✓ Space maintenance
✓ Stabilization of the blood clot
✓ Cell occlusiveness
✓ Mechanical strength
✓ Predictable resorption rate
✓ Easy to modify and manipulate
IDEAL CHARACTERISTICS OF MEMBRANE MATERIAL
Membranes used in GBR techniques may be divided into two categories: non-resorbable and resorbable. Non-resorbable membranes are bio-inert and require a second surgical procedure to remove after bone regeneration is complete. Resorbable membranes are naturally biodegradable and have varying resorption rates. However, whether non-resorbable or resorbable, all membranes differ in their biomaterial and physical characteristics, with associated advantages and disadvantages for various clinical situations. To determine which membrane material to use, consider that the ideal membrane should possess the following characteristics:
1. Tissue compatibility — Ideally, the membrane should be biocompatible, resulting in no inflammation or interaction between the membrane and the host tissue, to avoid wound dehiscence or infection.
2. Space maintenance — The membrane should have the ability to maintain space to allow for the bone regeneration process. Adequate membrane stiffness is paramount to maintain space and prevent defect collapse (Fig. 1).
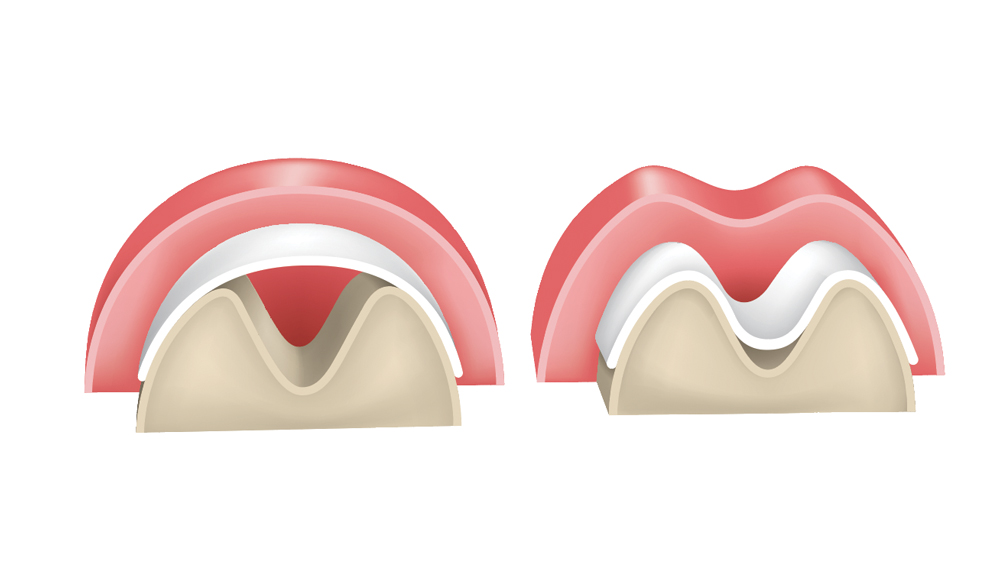
Figure 1: Membranes must exhibit space-maintenance qualities to allow bone regeneration to occur. If the membrane is not sufficiently stiff, there is potential for it to collapse within the defect, which results in altered bone growth.
3. Stabilization of the blood clot — The membrane should provide stabilization of the blood clot, which allows the regeneration process to progress and reduce connective-tissue integration into the defect.
4. Cell occlusiveness — The surface of the membrane should prevent fibrous tissue from invading the graft site, which directly correlates to the membrane porosity. A larger pore size may inhibit bone formation by allowing the overpopulation of faster-growing cells. When the pore size is too small, cell migration is limited for collagen deposition and the formation of avascular tissue results.
5. Mechanical strength — The membrane should have high durability and mechanical strength to protect the blood clot and resist passage of unwanted cells and bacteria.
6. Predictable resorption rate — The resorption time of the membrane should coincide with the regeneration rate of bone tissue, which is dependent on the location of the graft, vascularity and the quantity of graft material.
7. Easy to modify and manipulate — The membrane should be capable of size and shape alteration while maintaining adequate stiffness to prevent collapse of the graft site.
TYPES OF MEMBRANES
I. Non-Resorbable Membranes
Non-resorbable membranes exhibit excellent biocompatibility, superior mechanical strength and increased rigidity, and generally achieve more favorable space maintenance than resorbable membranes. However, wound dehiscence is more common with non-resorbable membranes, and these membranes have the disadvantage of the need for a second surgery, resulting in increased morbidity, costs and patient discomfort. The most common types of non-resorbable membranes include polytetrafluoroethylene (PTFE) and titanium mesh.
PTFE Membranes
PTFE membranes can be differentiated by expanded, high-density and titanium-reinforced forms:
a. Expanded PTFE membranes — The expanded PTFE membrane (e-PTFE) was the first type of membrane used in implant dentistry and was the gold standard for bone regeneration in the 1990s. The e-PTFE membrane is advantageous as it prevents fibroblasts and connective-tissue cells from invading the bone defect, yet allows the osteogenic cells to repopulate the graft area. The most common e-PTFE membrane in implant dentistry is GORE-TEX® (W.L. Gore & Associates, Inc.; Flagstaff, Ariz.).
The two sides of e-PTFE membranes have different features: one side is approximately 1 mm thick with 90 percent porosity, which impedes the growth of epithelium; and the other side is approximately 0.15 mm thick with 30 percent porosity, which provides space for new bone growth and prevents fibrous tissue ingrowth.4
The e-PTFE membranes have a high incidence of exposure, thereby resulting in an increased infection rate because of the ingrowth of bacteria into the highly porous structure. Additionally, the porous structure, with an approximate pore size of 5–20 micrometers, allows for soft-tissue ingrowth, leading to increased difficulty in removal.
b. High-density PTFE membranes — Because of the associated complications of e-PTFE membranes, a higher density material — less than 0.3 microns — was developed in the early 1990s under the name Cytoplast™ (Osteogenics Biomedical; Lubbock, Texas). This high-density PTFE (also termed dense PTFE or d-PTFE) was shown to have a lower risk of bacterial colonization in comparison to e-PTFE membranes, therefore resulting in fewer infections. These membranes are advantageous as they do not require soft-tissue closure because of their high density and small pore sizes (Fig. 2). The membrane prevents passage of bacteria while allowing oxygen diffusion and passage of small molecules. Because of lack of tissue ingrowth, d-PTFE membranes are much easier to remove.
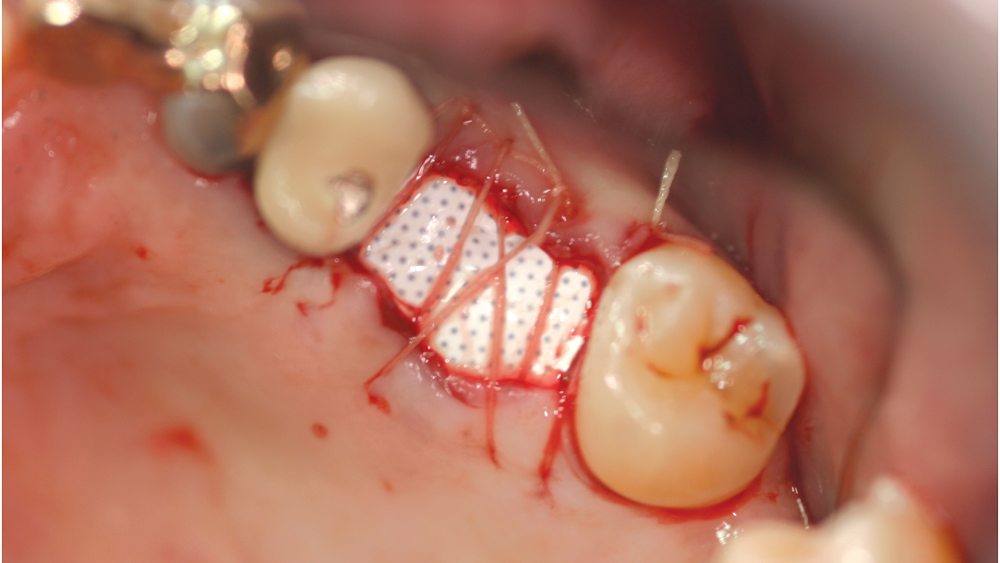
Figure 2: An advantage of high-density PTFE membranes is that they do not require soft-tissue coverage. However, these membranes do require removal after approximately 30 days.
c. Titanium-reinforced PTFE membranes — To increase the rigidity of e-PTFE and d-PTFE membranes, titanium was added to the PTFE membranes. Because of the increased structural rigidity, these membranes are easier to modify to conform to the defect. These types of membranes are especially useful in the treatment of large osseous defects (Fig. 3).
Titanium Mesh Membranes
Titanium mesh is a non-resorbable membrane that has been shown to be effective in maintaining space without collapsing. The membranes are flexible and can be bent and manipulated to form around the bony defect. The titanium mesh has demonstrated biocompatibility and features holes within the mesh, which allow for maintaining blood supply from the periosteum. The disadvantages of titanium mesh are increased wound dehiscence and difficulty in maintaining soft-tissue coverage (Figs. 4a, 4b). This may lead to increased infection and patient discomfort, which could lead to the necessity for early removal.
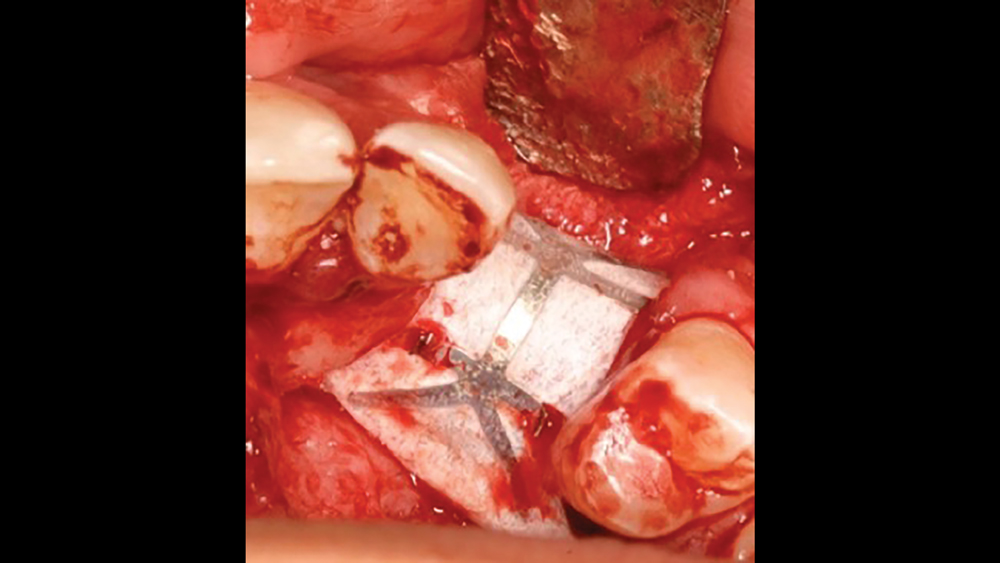
Figure 3: Titanium-reinforced PTFE membranes have increased rigidity compared to PTFE membranes without titanium, providing for enhanced space maintenance.
Titanium Mesh Membranes
Titanium mesh is a non-resorbable membrane that has been shown to be effective in maintaining space without collapsing. The membranes are flexible and can be bent and manipulated to form around the bony defect. The titanium mesh has demonstrated biocompatibility and features holes within the mesh, which allow for maintaining blood supply from the periosteum. The disadvantages of titanium mesh are increased wound dehiscence and difficulty in maintaining soft-tissue coverage (Figs. 4a, 4b). This may lead to increased infection and patient discomfort, which could lead to the necessity for early removal.
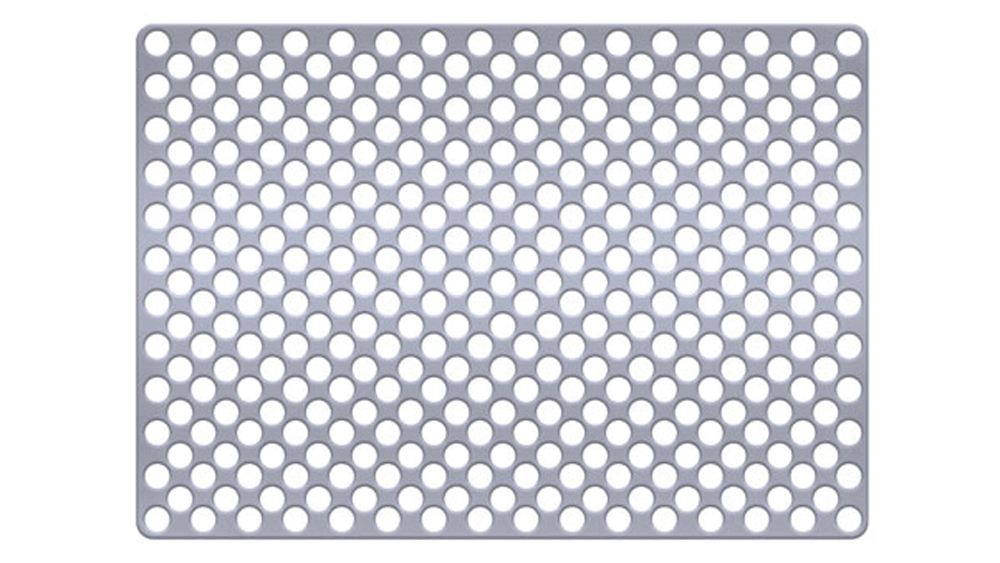
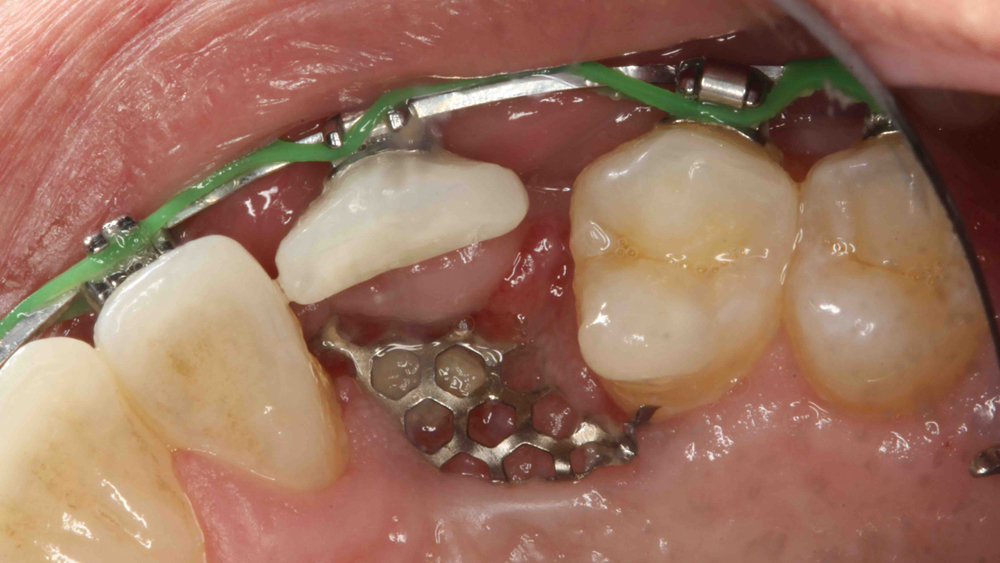
Figures 4a, 4b: Titanium mesh membranes have holes incorporated within the mesh (4a), which allow for maintaining blood supply; however, these membranes have the disadvantage of increased wound dehiscence, which commonly involves exposure of the mesh (4b).
II. Resorbable Membranes
Resorbable membranes exhibit the advantage of no second-stage surgery for removal, thus decreasing discomfort and morbidity to the patient. However, the drawbacks of collagen include an unpredictable resorption time, which may adversely affect the amount of bone formation. Resorbable membranes derived from xenogeneic collagen for use in GBR procedures are the most popular membranes utilized in implant dentistry today. The various types of resorbable membranes include collagen, pericardium, platelet-rich fibrin, and acellular dermal matrix.
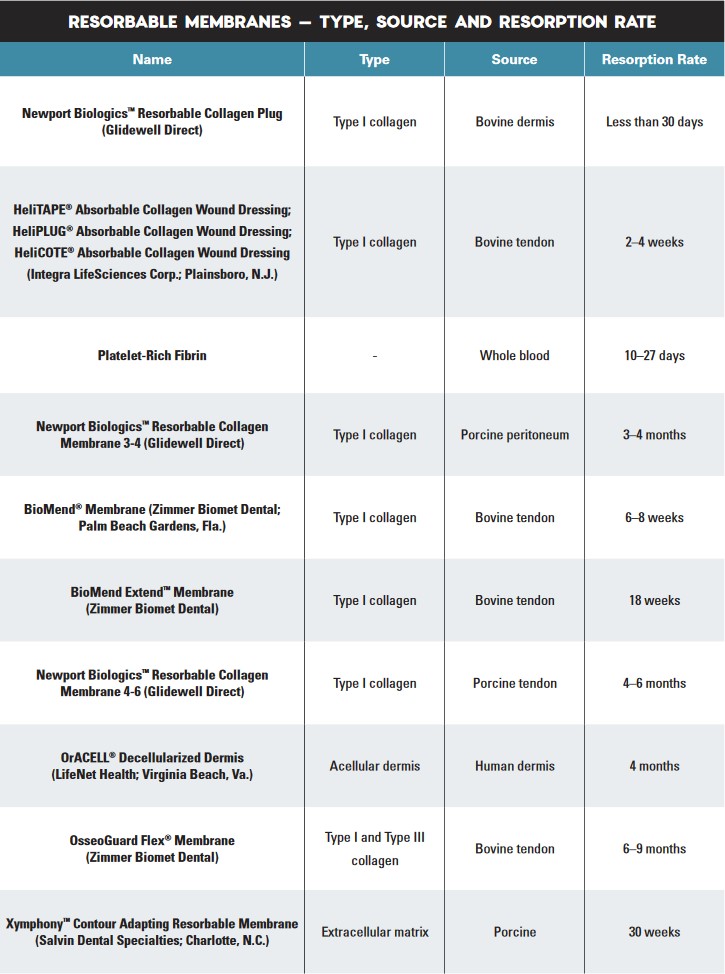
Collagen Membranes
Resorbable collagen membranes consist of either type I or type III collagen from bovine or porcine origin. Collagen membranes are easy to manipulate and have favorable effects on coagulation and wound healing, variable cross-linking, low antigenicity and high tensile strength.5 Additionally, they inhibit epithelial cells, promote the attachment of connective-tissue cells, and increase platelet aggregation, which leads to wound stabilization and increased healing.
Collagen constitutes over 50 percent of the proteins in the human body. As the collagen membrane is degraded through enzymatic reactions, the process resembles normal tissue turnover.6 Today, most collagen membranes are derived from allogenic or xenogeneic sources, which have become popular in implant dentistry.7 They act as scaffolding for osteoconduction, increase platelet aggregation and stability of clots, and allow for the attraction of fibroblasts for healing.8,9 Collagen membranes are manufactured with a variable resorption rate, which occurs through inflammatory cell biodegradation. The resorption rate is altered via the manufacturing process by the amount of cross-linking.10
Collagen barriers are available in various forms:
a. Collagen plugs are mainly used to control bleeding and maintain the blood clot within extraction sites (Figs. 5a, 5b). Collagen plugs are usually a soft, pliable, sponge-like material that rapidly absorbs blood, thereby creating an artificial clot. The collagen allows aggregation of platelets, which results in the degranulation and release of bone-growth factors. Collagen plugs have a resorption time of approximately 10–14 days.
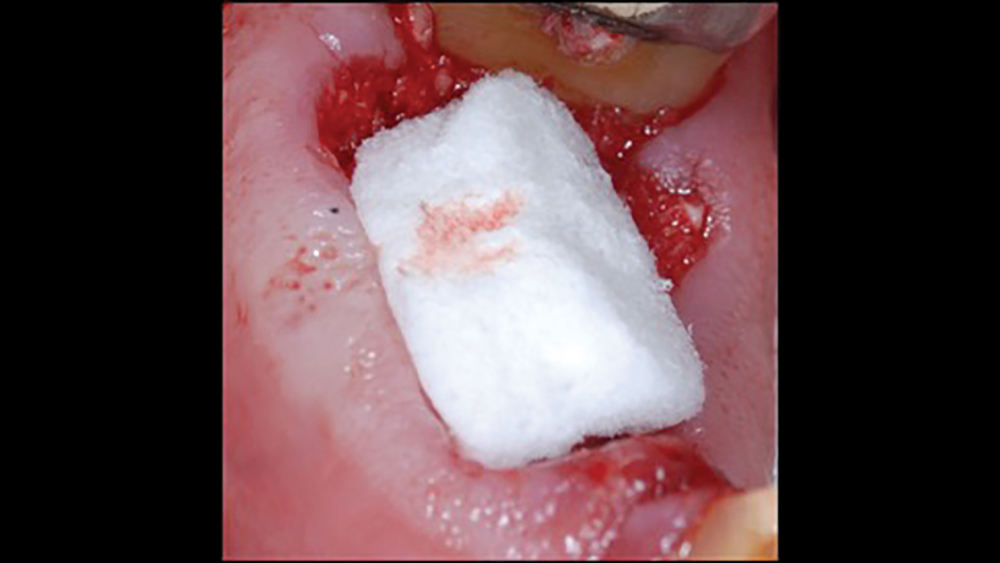
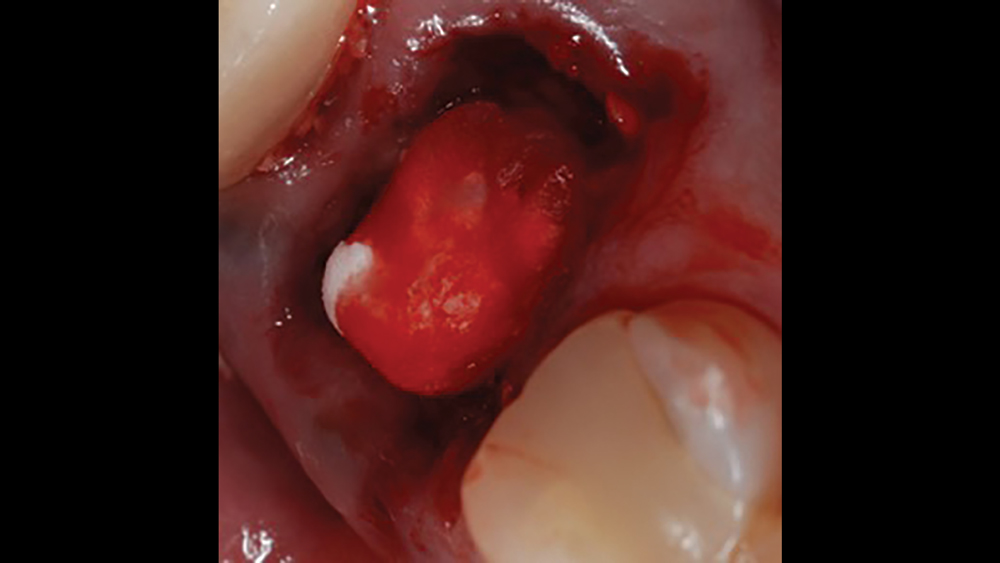
Figures 5a, 5b: Used to control bleeding and maintain the blood clot within the extraction site, the Newport Biologics™ Resorbable Collagen Plug (Glidewell Direct; Irvine, Calif.) supports predictable bone regeneration.
b. Collagen tape is a thin, pliable collagen used for hemostasis and small graft sites (Figs. 6a, 6b). Additionally, collagen tape is used for sinus graft procedures as the first-layer grafting technique.
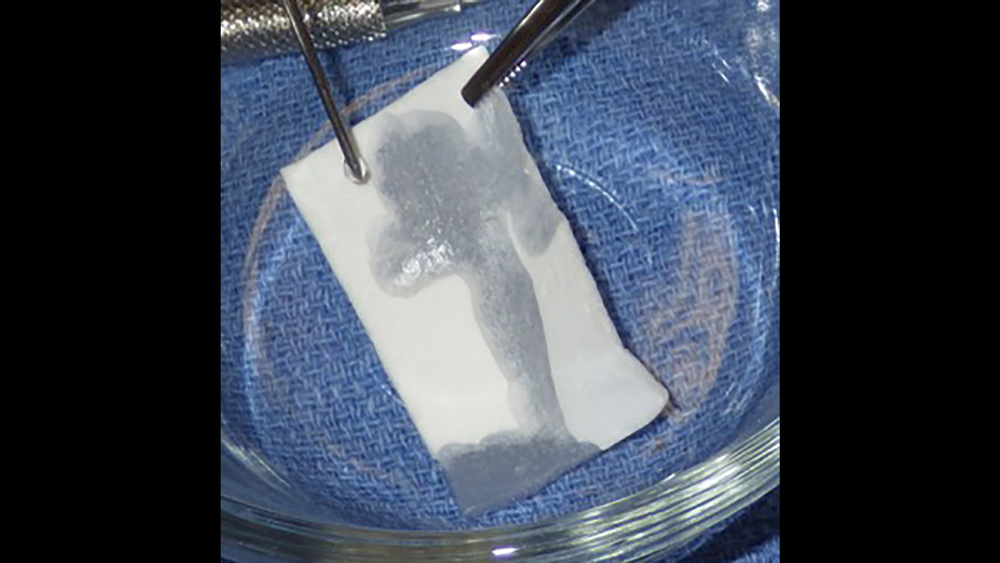
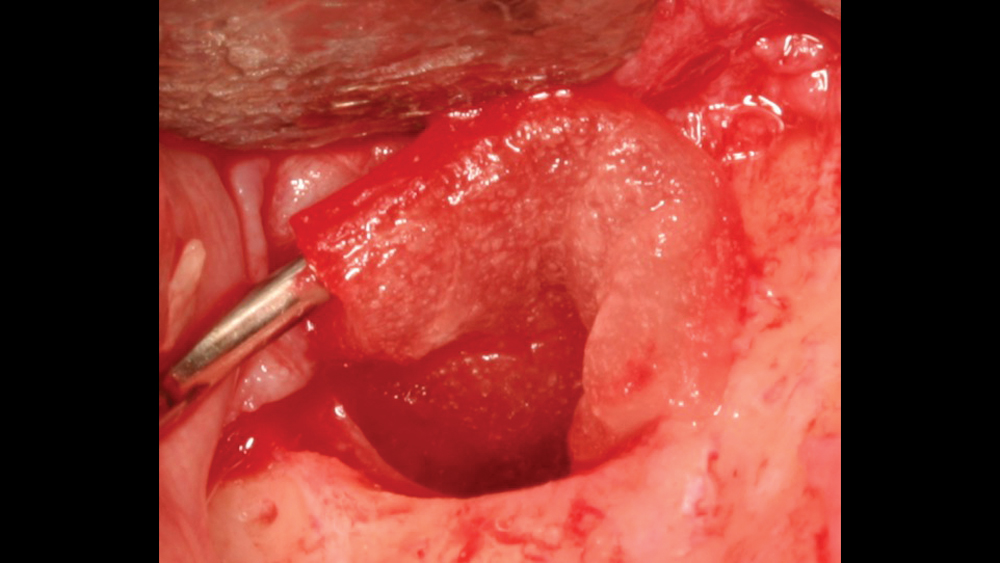
Figures 6a, 6b: Collagen tape may be used for hemostasis and small graft sites, or for sinus graft procedures as the first layer grafting technique.
c. Regular collagen membranes resorb in three to four months and are mainly used for guided bone regeneration for small- to medium-size bony defects (Figs. 7a, 7b). Ideally, primary closure is recommended to decrease graft morbidity.
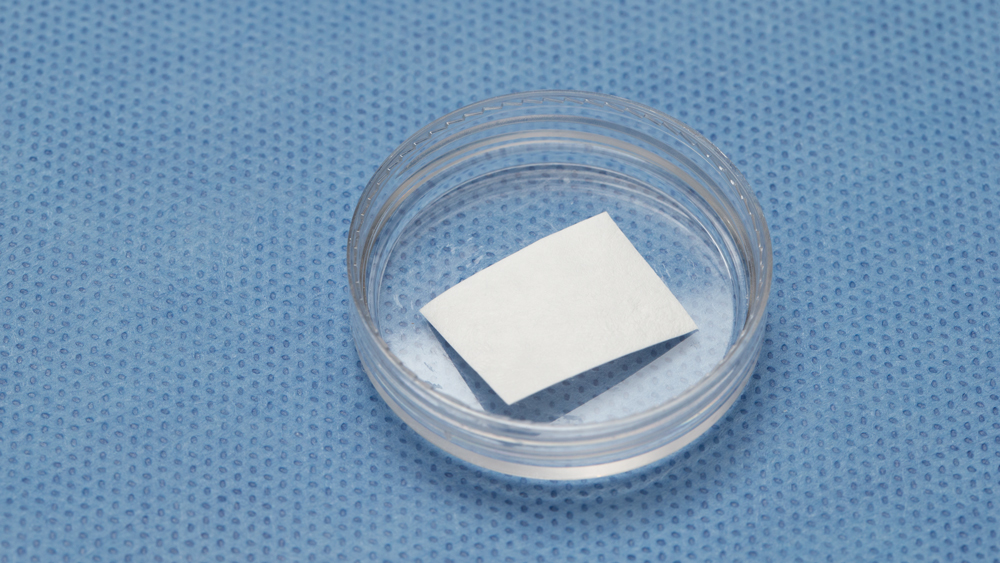
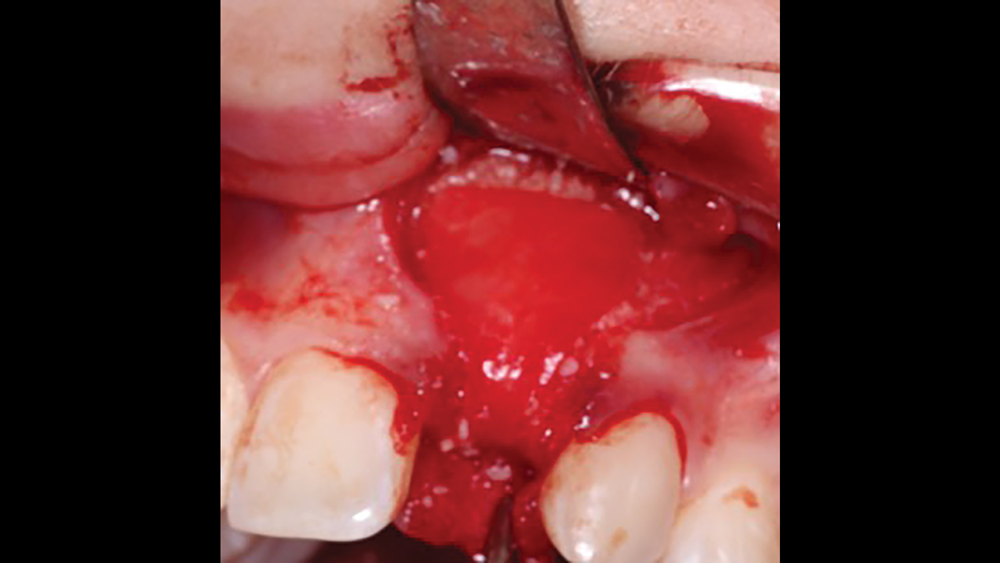
Figures 7a, 7b: A regular collagen membrane that resorbs in three to four months, such as the Newport Biologics Resorbable Collagen Membrane 3-4, is frequently used for guided bone regeneration for small- to medium-size bony defects.
d. Extended collagen membranes resorb in four to six months and are used for larger bony defects that require longer healing periods (Figs. 8a, 8b). These membranes are modified by increasing the cross-link density. However, longer-acting collagen membranes have been shown to have a greater host-tissue response, impaired vascularization, and tissue dehiscence.11
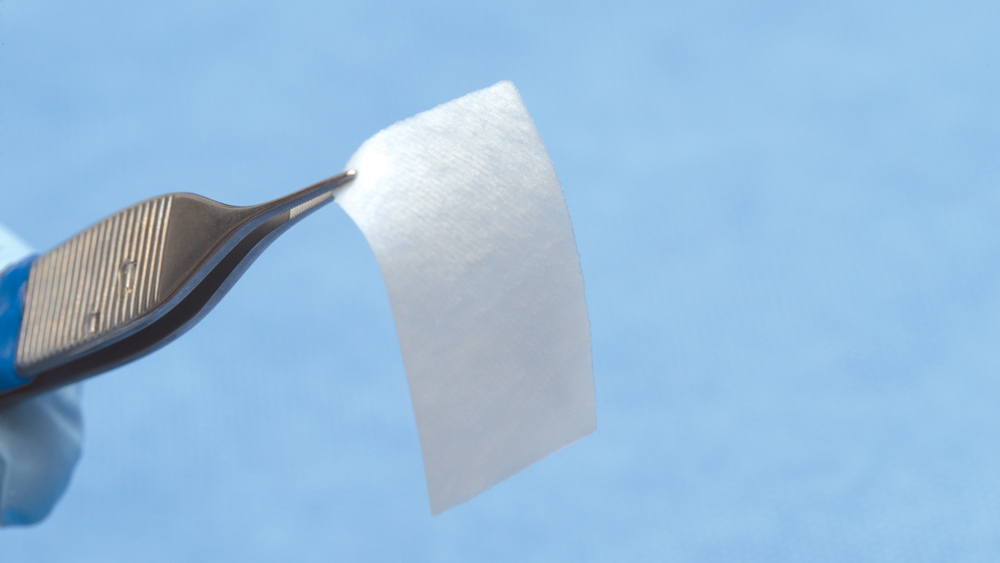
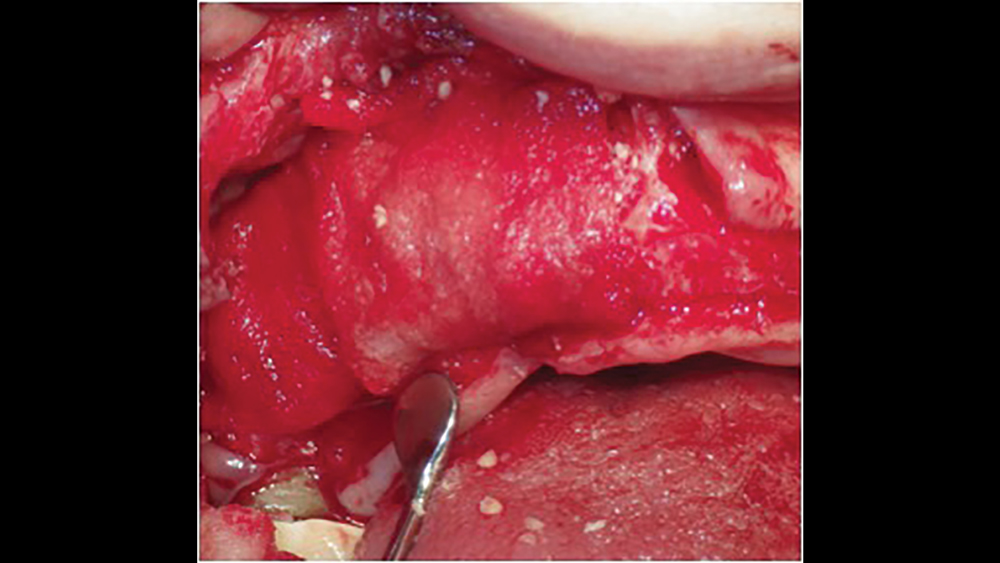
Figures 8a, 8b: Extended duration collagen membranes resorb in four to six months and are used for larger bony defects that require longer healing periods. For example, the Newport Biologics Resorbable Collagen Membrane 4-6 acts as scaffolding for osteoconduction, increases platelet aggregation and stability of clots, and allows for the attraction of fibroblasts for healing.
Although there are many advantages of collagen membranes, they have been shown to be problematic if exposed to the oral environment. When collagen membranes are exposed, this may result in bacterial growth and fibroblast migration, leading to an increased morbidity of the graft site. Additionally, collapse of the membrane may result, which compromises the space-maintenance qualities of the membrane.
Pericardium Membranes
The most common pericardium membranes are of either bovine or porcine origin, with bovine having a greater collagen content (Fig. 9). They generally consist of three layers with collagen and elastic fibers in an amorphous matrix. Their surface is porous, which allows for cellular attachment and proliferation, yet has an increased density for soft-tissue exclusion. Pericardium membranes have shown a prolonged resorption in comparison to collagen membranes.
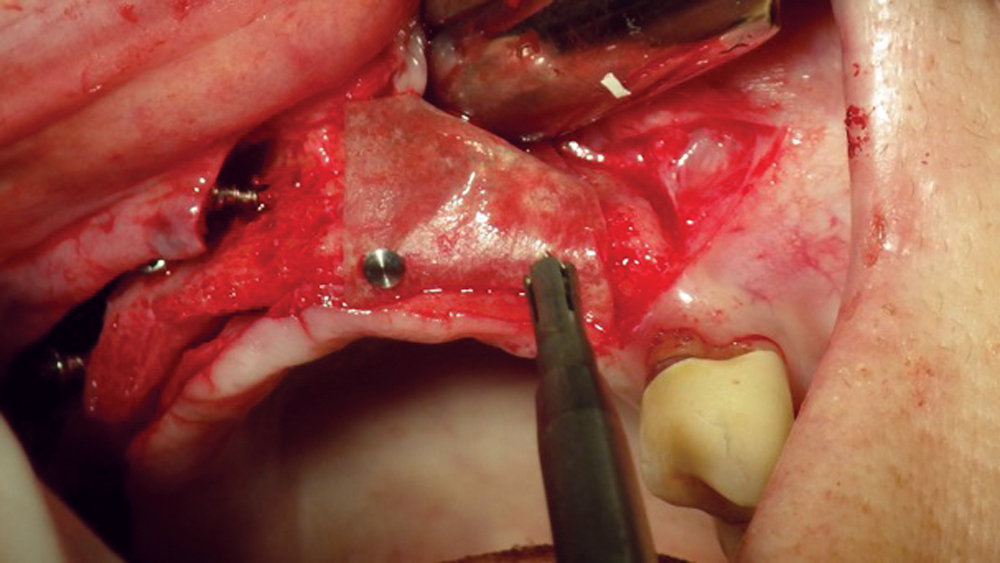
Figure 9: Pericardium membranes are typically derived from a bovine or porcine source.
Platelet-Rich Fibrin
The use of platelet-rich fibrin (PRF) is increasing in popularity for GBR procedures (Figs. 10a–10d). This second-generation platelet concentrate has the advantages of lower cost, no need for additional reactive agents, and a higher concentration of platelets in comparison to platelet-rich plasma. The PRF protocol involves the centrifuging of the patient’s whole blood, resulting in the formation of three layers. The bottom layer contains red blood cells, which is discarded; the top layer is clear-colored and is termed platelet-poor plasma; and the middle layer, which is the fibrin matrix, is used as a membrane in bone regeneration procedures.
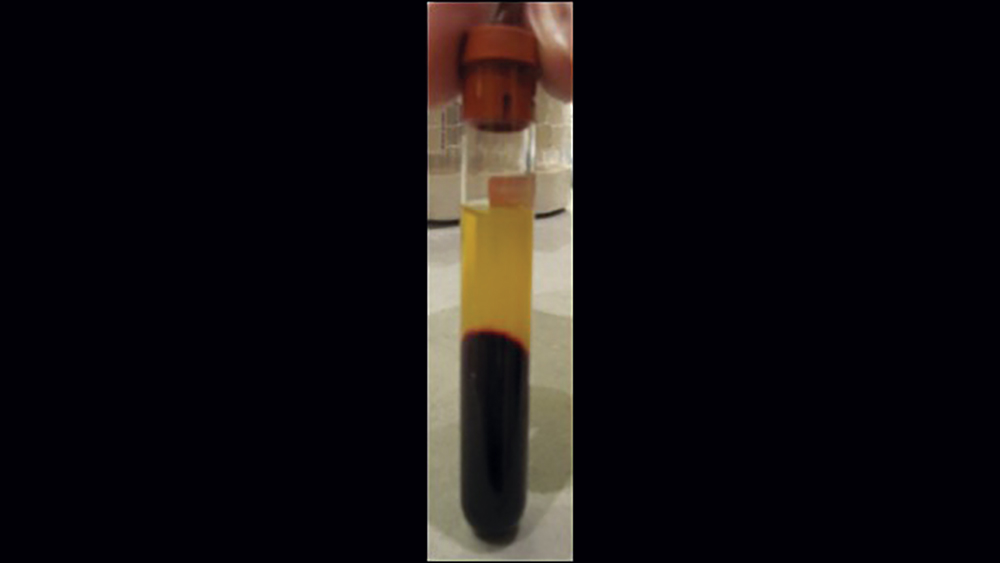
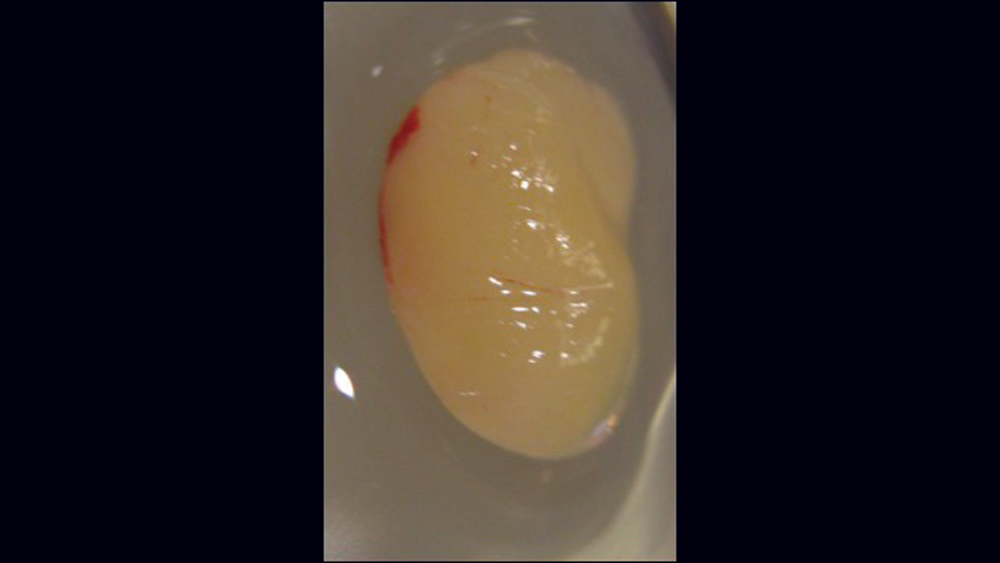
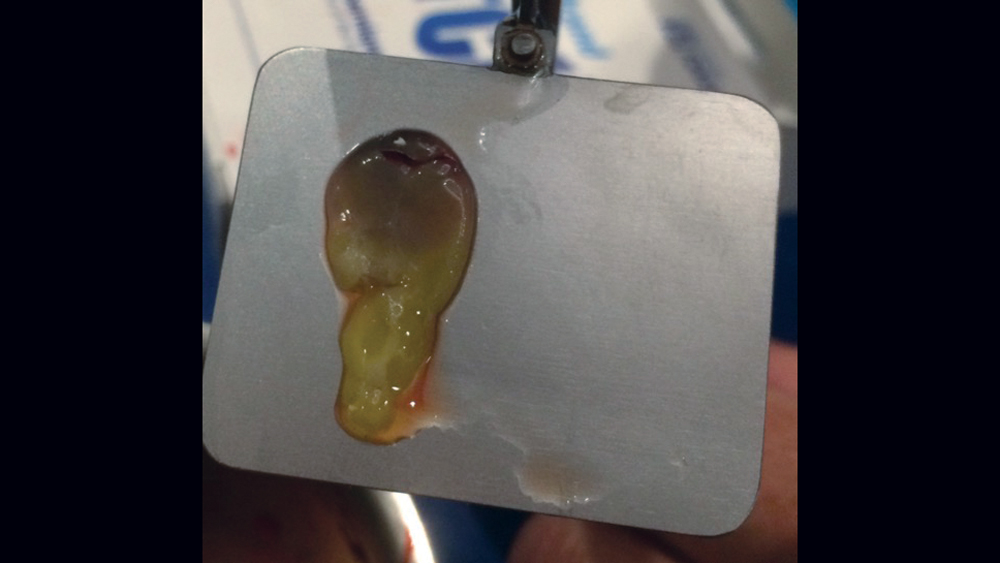
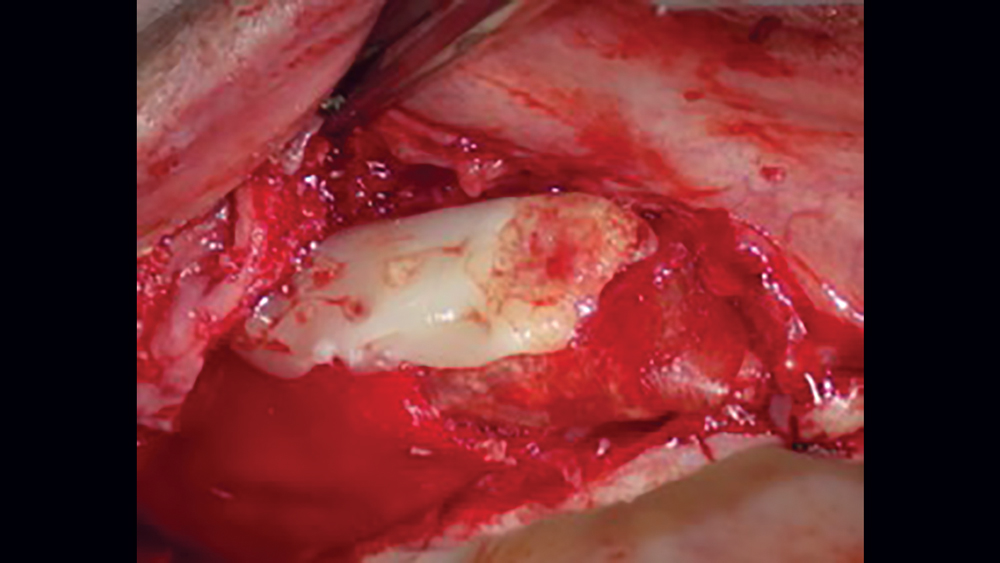
Figures 10a–10d: Platelet-rich fibrin has the advantages of lower cost, no need for additional reactive agents, and a higher concentration of platelets in comparison to platelet-rich plasma. Shown, a venipuncture was performed to obtain approximately 10 cc of blood (10a). The platelet-rich fibrin is obtained (10b), followed by modification of the fibrin clot into the membrane (10c), and then the PRF membrane is placed (10d).
Acellular Dermal Matrix
Acellular dermal matrix (ADM) is a biocompatible human (allograft) connective-tissue matrix derived through a process of removing all cells within the dermis. Because of the cells being removed during the manufacturing process, no viruses may be transmitted. Additionally, because of the acellular nature of this membrane, no inflammatory reactions or rejection will occur. The inert allograft, when used as a membrane, acts as an architectural framework that allows for fibroblast migration and vascularization (Figs. 11a, 11b).
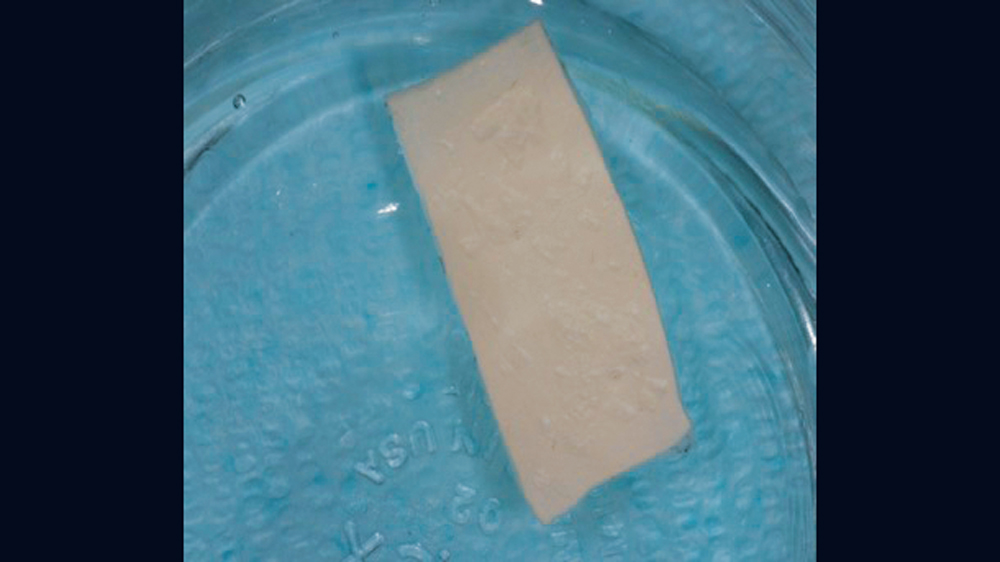
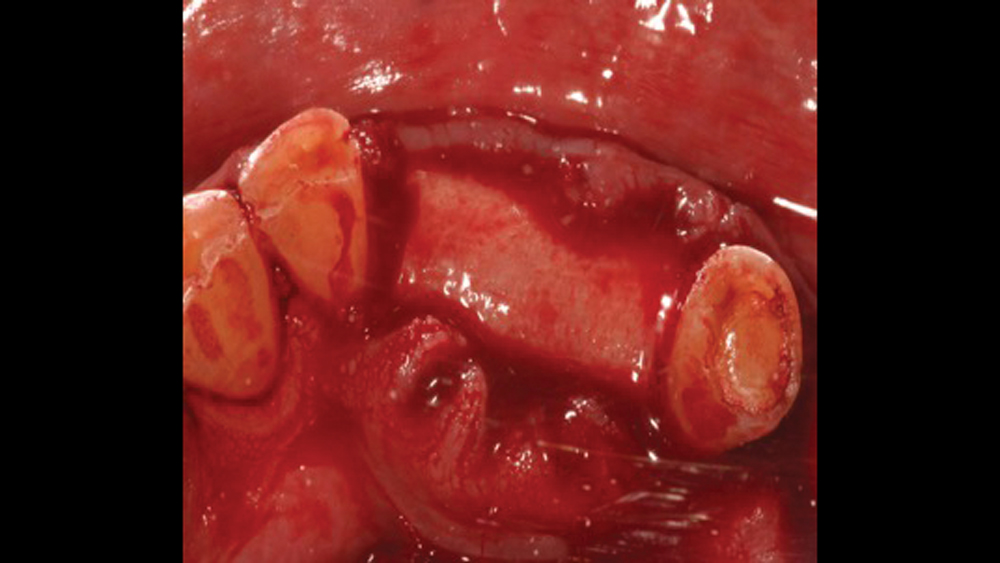
Figures 11a, 11b: Acellular dermal matrix is an inert allograft that may be used as a barrier membrane.
CONCLUSION
Membranes are a crucial and widely used component of implant dentistry. They act as a biological and mechanical barrier against the invasion of cells not involved in bone formation, allowing for the migration of slower-migrating bone-forming cells into the defect sites. Membranes are divided into non-resorbable and resorbable categories and are available in various forms. It is important for implant clinicians to determine which membrane to utilize based on different clinical situations as well as factors such as material composition and resorption time. With many options available, careful selection will support hard- and soft-tissue regeneration and ultimately increase the success rates of GBR procedures.
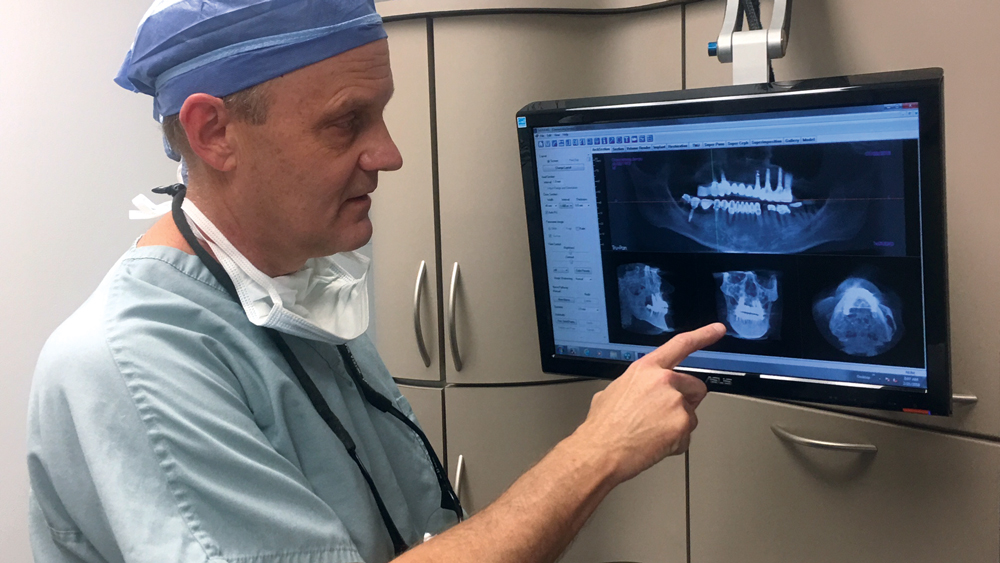
As bone defects heal, there is a competition between soft-tissue and bone-forming cells to invade the area. Membranes act as biological and mechanical barriers against the invasion of cells not involved in bone formation and allow for the migration of the slower-migrating bone-forming cells into the defect sites, providing for predictable bone regeneration.
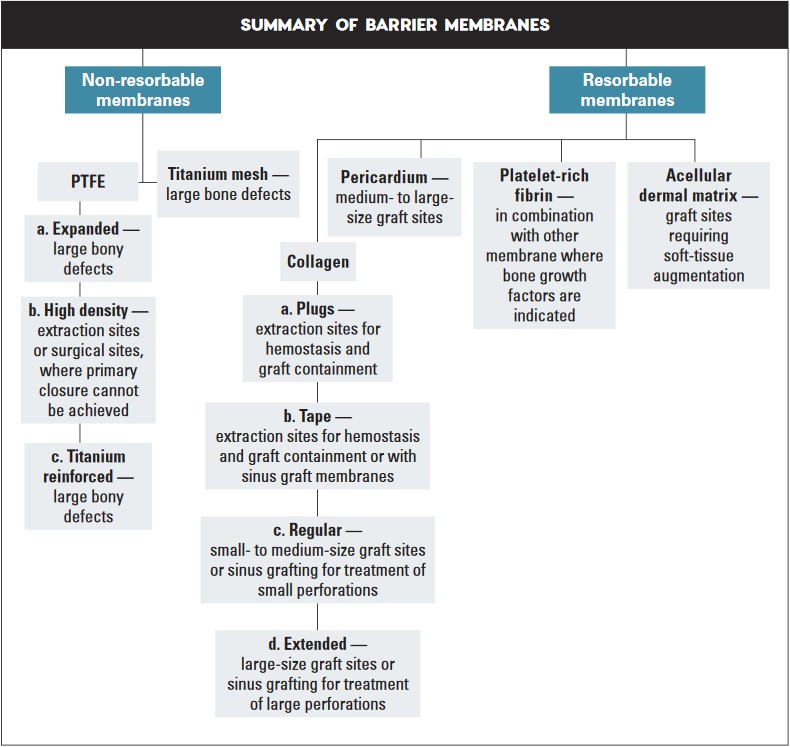
References
- ^Chou AH, LeGeros RZ, Chen Z, Li Y. Antibacterial effect of zinc phosphate mineralized guided bone regeneration membranes. Implant Dent. 2007 Mar;16(1):89-100.
- ^Nishibori M, Betts NJ, Salama H, Listgarten MA. Short-term healing of autogenous and allogeneic bone grafts after sinus augmentation: a report of 2 cases. J Periodontol. 1994 Oct;65(10):958-66.
- ^Pitaru S, Tal H, Soldinger M, Grosskopf A, Noff M. Partial regeneration of periodontal tissues using collagen barriers. Initial observations in the canine. J Periodontol. 1988 Jun;59(6):380-6.
- ^Zhang Y, Zhang X, Shi B, Miron RJ. Membranes for guided tissue and bone regeneration. Annals of Oral & Maxillofacial Surgery. 2013 Feb;1(1):10.
- ^ Pocket Dentistry [internet]. Barrier membranes for guided bone regeneration; [updated 2015 Jan 5; cited 2017 Dec 28]. Available from: https://pocketdentistry.com/3-barrier-membranes-for-guided-bone-regeneration/#end_en139.
- ^Rispoli L, Fontana F, Beretta M, Poggio CE, Maiorana C. Surgery guidelines for barrier membranes in guided bone regeneration (GBR). J Otolaryngol Rhinol. 2015;1(2):1-8.
- ^Almazrooa SA, Noonan V, Woo SB. Resorbable collagen membranes: histopathologic features. Oral Surg Oral Med Oral Pathol Oral Radiol. 2014 Aug;118(2):236-40.
- ^Luitaud C, Laflamme C, Semlali A, Saidi S, Grenier G, Zakrzewski A, Rouabhia M. Development of an engineering autologous palatal mucosa-like tissue for potential clinical applications. J Biomed Mater Res B Appl Biomater. 2007 Nov;83(2):554-61.
- ^Yamada M, Kubo K, Ueno T, Iwasa F, Att W, Hori N, Ogawa T. Alleviation of commercial collagen sponge- and membrane-induced apoptosis and dysfunction in cultured osteoblasts by an amino acid derivative. Int J Oral Maxillofac Implants. 2010 Sep-Oct;25(5):939-46.
- ^Rothamel D, Schwarz F, Sager M, Herten M, Sculean A, Becker J. Biodegradation of differently cross-linked collagen membranes: an experimental study in the rat. Clin Oral Implants Res. 2005 Jun;16(3):369-78.
- ^Schwarz F, Rothamel D, Herten M, Wüstefeld M, Sager M, Ferrari D, Becker J. Immunohistochemical characterization of guided bone regeneration at a dehiscence-type defect using different barrier membranes: an experimental study in dogs. Clin Oral Implants Res. 2008 Apr;19(4):402-15.